摘要
青藏高原是生态系统响应全球变化研究的热点区域。目前,对该区域水生生物群落如何响应气候变化和人类活动的认识还存在不足,需要更多的研究案例。本研究以古湖沼学手段研究了青藏高原东南部的兴伊措过去300年来的湖泊生态系统演变历程。研究结果显示,1930年之前沉积钻孔中生物指标硅藻以及理化指标变化不大,表明湖泊及其流域环境变化较为有限。1930年以来,该湖泊流域风化和侵蚀持续增加,湖泊营养水平上升。研究时间段内,硅藻群落中优势属种变化不明显,但稀有硅藻属种在1930年后出现显著变化。该研究认为,气候变化是兴伊措流域风化、侵蚀增加的主要原因,人类放牧活动可能加剧了气候变化的影响。当前的环境变化尚未对兴伊措水生生态系统优势类群造成显著影响,但湖泊中稀有属种自1930年以来的快速响应表明如果环境压力持续增加,未来该区域湖泊生态系统可能面临突变风险。
Abstract
Tibetan Plateau is particularly sensitive to climate change and is a hotspot for studying ecosystem responses to global change. Currently, there is much controversy surrounding studies of aquatic biota response to climate change in this region, requiring further investigation. In our study, the evolution of the lake ecosystem over the last 300 years was investigated by paleolimnological methods in Lake Xingyicuo on the southeastern Tibetan Plateau. The results showed that the lake and surrounding environment had undergone limited changes before 1930. Since 1930, weathering and erosion had increased in the area leading to increased nutrient levels in the lake. Principal component analysis revealed significant ecological changes in rare diatom species after 1930, while the dominant species remained stable. Our study indicated that climate change was the primary cause of increased weathering and erosion in the Lake Xingyicuo watershed, with human grazing activities having a possible exacerbating effect. Although the current environmental changes have not yet caused significant changes in the aquatic ecosystems, our studies found rare species in the lakes responded quickly to climate changes since the 1930s. In the future, the lake ecosystems in this region may face a risk of rapid change if environmental pressures continue to increase.
Keywords
青藏高原被称为世界第三极或亚洲水塔[1],具有影响区域乃至全球气候的潜力[2]。近几十年来,气候变暖和人类活动(如放牧)[3]已经造成该区域永久冻土融化[4]、冰川后缩[5]以及土地覆盖的变化[6]。灌木植被在高原上分布较广,是土壤和水土保持的重要组成部分。由于变暖和干旱的压力持续增加,青藏高原灌木群落在1930年左右达到了临界点,覆盖度迅速下降[7]。该地区灌木的退化可能会增加湖泊流域的风化和侵蚀[8],但目前尚不清楚这一过程如何影响湖泊生态系统中的生物群落。
土壤侵蚀被认为对土地生产力、下游水质和生物地球化学循环有重大影响[9],这些过程可以改变湖泊环境和生物群落[10-11],甚至可能导致湖泊早期的富营养化[12]。硅藻作为水生生态系统中最重要的初级生产者之一,对土壤侵蚀有关的水环境因素(pH值、氮磷负荷、盐度)高度敏感[13]。硅藻的硅质壳体可以在湖泊沉积物中长时间保存,是在长时间序列上研究生态系统对全球变化响应的良好代用指标[14]。对青藏高原东南部湖泊硅藻的古生态研究表明,过去100~200年以来,一些湖泊(如黑海、错恰湖、措木及日湖)硅藻组合由于气候变暖以及湖泊集水区的放牧活动发生了显著的变化[15-19]。这些湖泊硅藻群落的变化与北极地区大量湖泊中的变化非常相似,均从底栖类群(大型Aulacoseira和小型Fragilaria)转变为浮游类群(小型Cyclotella)和附生类群[20-22]。
然而,对青藏高原地区其他湖泊(例如月亮湖、沙德措、吉仁措、天才湖、伍须海、LC6湖、尕尔乌克湖和巴松措)的研究表明,在同一时期内它们的硅藻群落组成变化很小[17-18,23-26]。青藏高原东南部不同湖泊的硅藻群落对环境变化反应的显著差异可能源于其复杂的山地环境以及温度和湿度模式[27-28]。迄今为止,对青藏高原地区硅藻如何响应全球变化尚无统一的认识,可能需要更多的案例研究。
海子山地区分布有青藏高原东南部最大的冰川遗迹,冰川作用形成了众多湖泊。海子山位于季风区边缘,是气候变化的敏感区域。本研究选取了海子山地区最大的湖泊兴伊措作为研究对象,采集了湖泊沉积物岩芯,通过分析生物指标硅藻、理化指标和现代观测数据,以期能更加全面地了解过去300年以来气候变化和人类活动相互作用对该区域湖泊和流域产生的影响。本文重点关注的问题是气候变化和人类活动对兴伊措产生了哪些影响,以及硅藻群落如何响应这些环境变化。对该区域硅藻群落长期响应的研究有助于了解全球变化对该区域湖泊生态系统的影响。
1 材料与方法
1.1 研究地点
兴伊措位于青藏高原东南部的海子山地区,海拔4420 m,湖泊总面积约7.2 km2(图1),流域面积为70.72 km2。它由古冰体侵蚀堆集形而成,平均深度在3 m左右,最深处可达10 m。水体营养水平较低,总氮(TN)和总磷(TP)浓度分别为0.22和0.006 mg/L。兴伊措有河流的注入和流出,属于开口型淡水湖泊(图1b)。湖泊周围地形平坦,由草地和灌木覆盖,是当地的牧场之一。该区域年均温为4.6℃,年均降水量为651.3 mm。雨季集中在6—9月,降水量占全年的86%;旱季集中在10月—次年5月,降水量占全年的14%。
1.2 文献资料收集
图1中数字高程模型(DEM)数据来源于USGS(https://earthexplorer.usgs.gov/),青藏高原边界数据来源于http://www.geodoi.ac.cn。区域1901年以来的年平均气温数据(图2a)来自气候变化知识门户网站(https://climateknowledgeportal.worldbank.org.)。同时为了解过去3个世纪海子山地区的气候变化状况,本研究引用了基于青藏高原东南部树木年轮重建的相对湿度[29]和降水[30]数据(图2b)。四川省的氮肥数据取自四川省统计年鉴(图2c)。人口和牲畜数据(图2d)收集自甘孜州统计信息网(http://tjj.gzz.gov.cn/gzztjj/)和稻城县志。
图1兴伊措及其流域位置:(a)兴伊措流域地形图;(b)兴伊措卫星影像及采样点位置;(c)本研究中提到的兴伊措及其他湖泊在青藏高原东南部的位置
Fig.1Location of Lake Xingyicuo and its watershed: (a) Topographic map of the Xingyicuo watershed; (b) Satellite image of Lake Xingyicuo and location of sampling sites; (c) Location of Lake Xingyicuo and other lakes mentioned in this study in the southeastern Tibetan Plateau
1.3 采样、沉积柱测年及理化指标分析
2020年夏季,使用奥地利UWITEC重力采样器从兴伊措湖心(30°10′N,100°37′E,水深10 m处)采集1根62 cm长的沉积物岩芯。现场以0.5 cm厚度进行分样,共获得124个沉积物样品,存放于塑封袋中,冷藏在低于4℃的环境以备实验室分析。沉积物定年采用210Pb、226Ra和137Cs定年方法,使用EG&G Ortec生产的高纯度锗井型探测器(HPGe GWL-120-15)测定其比活度。沉积物年龄的计算采用恒定供应率(CRS)模式[31]。通过使用该岩芯平均沉积速率推算得出210Pbex基底以下的年代。总有机碳(TOC)和总氮(TN)使用Euro Vector生产的EA3000元素分析仪进行测定。利用电感耦合等离子体原子发射光谱仪(ICP-AES)测定沉积物中的元素钙(Ca)、钾(K)、铝(Al)、钛(Ti)、磷(P),并将测试误差控制在±5%以内。利用英国Malvern公司生产的Mastersize3000激光粒度分析仪分析粒度。
1.4 硅藻分析
硅藻的制备过程遵循Battarbee等[32]的方法。取约0.3 g干样,放入50 mL离心管中,在60℃水浴条件下先后加入过量的10% HCl和30% H2O2以完全去除碳酸盐和有机质。在处理后的样品中加入蒸馏水至40 mL,静置48 h后倒掉上清液,如此重复3次以上使溶液达到中性,利用振荡将清洗后的样品均匀混合,用移液枪准确吸取 100 μL溶液滴至盖玻片上,待自然风干后制成永久样片。硅藻的计数和鉴定过程在OLYMPUS BX-53光学显微镜的油镜下进行(放大倍数 100×10)。沉积物样品鉴定硅藻壳体不少于500 粒。根据Krammer和Lange-Bertalot(1986—1991年)[33]的分类系统进行属种鉴定,并将鉴定结果转化为每个属种的相对丰度以分析。硅藻属种学名使用Algaebase(www.algaebase.org)进行更新。以上测试分析过程均在中国科学院南京地理与湖泊研究所湖泊与环境国家重点实验室完成。
1.5 数值分析
首先利用平方根变换对硅藻相对丰度数据进行标准化,接着进行排序分析。标准差<2,因此采用主成分分析(PCA)研究硅藻群落的主要演变趋势。分别对所有分类群(106种)和相对丰度低于5%的稀有属种(96种)作PCA分析(将相对丰度小于5%的硅藻属种定义为稀有属种),对比了PCA第1轴(PCA1)得分和第2轴得分(PCA2)在所有属种和稀有属种中的变化趋势,以研究环境变化对不同类型属种的影响。同时计算出Hill N2指数代表多样性以反映硅藻群落物种多样性随时间的变化趋势。上述所有分析均在Canoco 5中进行[34]。本研究使用Tilia绘制硅藻相对丰度图谱[35],并使用其内置的地层约束聚类分析方法——累计增量平方和(CONISS)进行生物地层划分。利用R语言 “vegan” 程序包[36]计算时间序列中相邻两个样品的β多样性指数(βsor)。
2 结果
2.1 气候与统计数据
自1901年以来,该地区的平均温度显著上升,1930—1960年间出现一个显著的暖期(图2a)。树轮重建的气候数据显示,自1700年以来青藏高原东南部的相对湿度和降水量呈下降趋势(图2b)。1949年以来,四川省的年化肥使用量快速增长(图2c)。稻城县的人口自1949年以来稳步增长,到2020年几乎翻倍。同期,稻城县积极引进新的牲畜品种并改善草场管理,这促进了畜牧业的发展。1949—2000年,牲畜数量增加了近3倍(图2d)。放牧数量的快速增长造成草场超量载畜、饲草不足。2000年后稻城县启动“退牧还草”工程后,放牧数量开始下降(图2d)。
2.2 沉积钻孔定年
从图3可以看出,兴伊措沉积岩芯中的137Cs比活度表现为从底部到顶部先升高后下降的趋势,11.5 cm处有明显的峰值。210Pbex随深度呈指数递减,大约在24.5 cm处达到平衡。因此样品的年代计算采用恒定供应率模型(constant rate of supply,CRS)[31],年代—深度结果如图3d所示。CRS模型计算的年代可以和1963年的137Cs峰值相对应(图3c、d)。CRS模型得出的沉积速率约为0.04 g/(cm2·a)。自1930年以来,沉积速率显示出增加趋势,并在2000年后开始放缓(图3d)。24.5 cm处的沉积柱年代约为1877年,24.5 cm以下的沉积柱年代通过平均沉积速率外推获得,沉积柱底部样品的年代约为1702年。
2.3 地球化学、物理指标
根据硅藻分区并结合其变化趋势划分兴伊措沉积钻孔理化指标图中的3个分区。该图显示沉积物TOC和TN的变化趋势相似,1930年之前稳定,之后迅速增加。C/N比值在1930年之前稳定在10.6左右,之后下降到8左右。TP在1930年之前呈轻微下降趋势,1930—1990年快速增加,1990—2020年相对稳定。沉积物中值粒径在1820—1875年间出现较大的波动,在1930—2000年间大幅增加,粒径从12 μm左右增大至20 μm左右,2000—2022年间粒径从20 μm减小到16 μm左右(图4)。1930年之后沉积速率也呈上升趋势,从0.02 g/(cm2·a)上升到0.6 g/(cm2·a),但在2000年之后出现小幅下降趋势,下降至0.5 g/(cm2·a)左右(图4)。
Ca/K比值在1830年之前缓慢增加,1830—1930年变化不大,1930—2020年快速增加(图4)。K/Al和K/Ti在1930年之前变化不大,在此之后快速增加。总体而言,营养元素、地球化学元素、粒径和沉积速率在1930年之前变化较小,之后则发生了显著变化。
2.4 化石硅藻
整个岩芯共鉴定出硅藻21属106种,主要属种为浮游硅藻Cyclotella cyclopuncta,相对丰度占40%左右。其次是底栖类群Achnanthidium minutissimum,相对丰度约为20%,Staurosira construens、Platessa holsatica和Aulacoseira ambigua的相对丰度都为5%左右(图5)。CONISS聚类分析确定了硅藻群落时间序列上的两个组合带。在组合带1(1930年前),硅藻组合并没有明显变化,PCA1和PCA2得分保持稳定。Hill N2多样性在1702—1750年间呈现下降趋势,1750年之后呈现波动上升趋势。βsor在1730—1830年间有所下降,在此之后波动上升。相比于组合带1,组合带2(1930年以来)的主要属种并无显著变化,但所有属种的PCA2得分以及稀有属种的PCA1(<5%)得分在组合带2有了明显变化,相同的变化趋势表明PCA2得分主要反映了稀有属种的变化。一些稀有属种相对丰度在组合带2出现减少的趋势,这些属种(Eunotia arcus、Tabellaria flocculosa、Encyonema silesiacum、Navicula rodiosa)在弱酸性和贫营养生境中常见。Hill N2和βsor在组合带2呈现波动上升趋势。

图2研究区的气候记录和人类活动:(a)1901—2020 年区域年均气温(气候变化知识门户网站, https://climateknowledgeportal.worldbank.org/);(b)根据树木年轮重建的青藏高原东南部相对湿度和降水量数据[29-30];(c)1953—2007 年四川省氮肥年使用量(四川省统计年鉴);(d)1949—2020 年稻城县的人口和牲畜数量(甘孜州统计信息网和稻城县志)
Fig.2Climate records and human activities in the study area: (a) Regionally monitored mean annual temperatures from 1901 to 2020 (https://climateknowledgeportal.worldbank.org/) ; (b) Relative humidity and precipitation data in the southeastern Tibetan Plateau reconstructed from tree annual rings[29-30]; (c) Annual nitrogen fertilizer use in Sichuan Province from 1953 to 2007 (Sichuan Statistical Yearbook) ; (d) Population and livestock numbers in Daocheng County from 1949 to 2020 (Ganzi Statistical Information Network and Daocheng County Record)
3 讨论
过去3个多世纪以来,兴伊措经历了气温的上升和降水的减少,区域内人口迅速增长(图2d),畜牧业快速发展(图2d),湖泊受到气候变暖以及人类活动的影响。1930年以来湖泊营养快速增加,同时地球化学元素、粒度也发生了较大的变化。硅藻主要属种在过去300年来保持稳定,但相对丰度小于5%的稀有属种在1930年之后发生较大变化。湖泊生态系统很可能受到气候和人类活动的共同影响。

图3兴伊措沉积钻孔210Pb和137Cs比活度与深度及年代深度模型:(a)210Pb与226Ra比活度随深度变化趋势;(b)210Pbex比活度随深度变化趋势;(c)137Cs比活度随深度变化;(d)CRS模型年代和沉积速率
Fig.3210Pb and 137Cs specific activities versus depth and chronological depth modeling for the sedimentary core from Lake Xingyicuo: (a) 210Pb and 226Ra specific activity trends with depth; (b) Trend of 210Pbex specific activity with depth; (c) 137Cs specific activity variation with depth; (d) CRS model ages and sedimentation rates

图4兴伊措沉积钻孔理化指标随深度变化趋势
Fig.4Trend plot of physicochemical indicators with depth for sedimentary cores from Lake Xingyicuo
3.1 气候变化和人类活动驱动了湖泊及其流域的环境变化
基于树轮重建和监测的气候数据显示[29-30],该地区在1930年后经历了气温升高和降水减少的过程。这可能导致冻土融化、高山草甸退化和土壤有机质的减少[37],从而造成流域风化、侵蚀的增加。在湖泊沉积物岩芯中,沉积物元素Ca/K的变化可用来推断过去风化强度的变化[38],因为在风化溶解时Ca在沉积物中更易形成,K则较为稳定[38-39]。自1930年以来兴伊措Ca/K增加反映了流域风化强度的持续增加(图4)。此外,元素的K/Al和K/Ti通常用来指代伊利石/高岭石比值的变化,伊利石是物理风化占主导地位的干旱地区更为常见的风化产物,高岭石是强烈化学风化的产物,其比值的变化可以反映物理和化学风化的强弱关系[40-41]。自1930年以来兴伊措K/Al和K/Ti的增加反映出流域气候趋于干燥、土壤发育不良,流域物理风化呈增加趋势(图4),这与该区域气候数据反映出的自然环境变化趋势较为一致(图2a、b)。
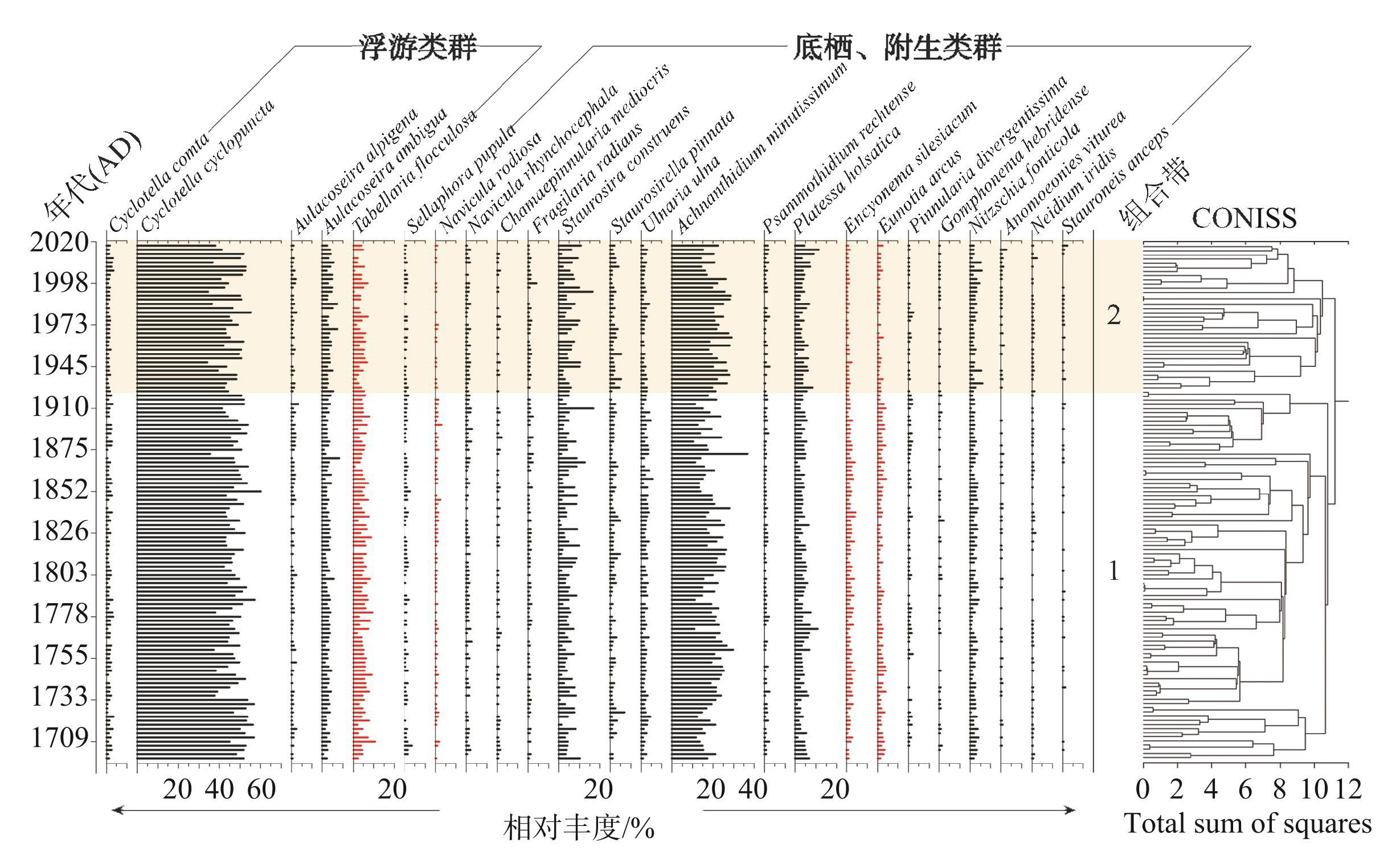
图5兴伊措沉积钻孔硅藻图谱(相对丰度下降的稀有属种以红色突出显示)
Fig.5Sedimentary core diatom profile of Lake Xingyicuo (rare genera with decreasing relative abundance are highlighted in red)
气候变化导致的流域内风化增加会促进侵蚀作用[42-43]。近几十年来,稻城县放牧活动增加使得流域内的植被覆盖率降低[44]。兴伊措自1930年以来,中值粒径和沉积速率均显示出增加趋势(图4),反映出该阶段流域侵蚀的增加,更多的碎屑物质进入湖泊。沉积速率和中值粒径在2000年后均有所下降(图4),可能与这一时期放牧活动的减少有关(图2b)。因此,人类放牧活动可能是流域侵蚀的影响因素之一。由于数据不足,本文无法区分人类活动和气候变化因素的相对贡献大小。
3.2 流域侵蚀增加导致湖泊环境和硅藻群落的变化
自1930年以来,兴伊措沉积物中的TN和TP迅速增加(图4),这与流域风化、侵蚀持续增加的时间较为一致。TN的增加也可能受到大气氮沉降的影响[17],但该地区氮肥的使用在1949年后才开始迅速增加(图2c),导致当前较高的氮沉降速率[45],氮沉降可能只占兴伊措TN来源的较小部分。自1930年以来,兴伊措的C/N从11降至8左右(图4)。该阶段气温呈现出相对较高的峰值(图2a),同时湖泊营养增加(图4),这造成湖泊内藻类大量繁殖,内源有机质比例增加[46],从而导致C/N下降。这一时期TOC增加了近2倍(图4)。
过去300年以来硅藻群落PCA1得分变化较小,但PCA2和PCA1(<5%)得分在1930年之后发生了显著变化(图6),这表明湖泊环境的变化对湖泊中的稀有属种产生了重大影响。这些属种包括Tabellaria flocculosa、Encyonema silesiacum、Eunotia arcus等,它们对水体营养和pH的变化较为敏感[47-48]。1930年以来,气候变化和人类活动导致流域植被覆盖度减少,使得陆源有机质的输入减少,而陆源碎屑颗粒输入增加。水体pH可能由陆源溶解有机碳(DOC)输入较多的弱酸性环境向陆源碎屑输入多的中性或弱碱性生境转变[48],从而导致一些稀有属种相对丰度的减少。βsor反映了兴伊措过去300年内硅藻群落组成沿时间梯度的差异性。βsor的整体上升趋势(图6)表明相邻时间序列上的硅藻群落组成差异逐步增大。然而,硅藻Hill N2多样性的增加(图6)表明,一定程度的环境干扰对于生物多样性具有促进作用[49]。总体来看,兴伊措及其流域环境发生了较大变化,但对硅藻群落的影响较为有限。
3.3 硅藻群落的变化表明区域生态变化的风险增加
以往对该地区大多数湖泊的古生态学研究发现,硅藻群落并没有显著变化,包括月亮湖[17]、沙德措[17]、吉仁湖[18]、天才湖[18]、伍须海[25]、LC6湖[25]、尕尔乌克湖[24]和巴松措[23](图1c)。本研究也发现在过去300年中,优势硅藻属种没有显著变化。从青藏高原东南部大区域来看,虽然湖泊环境和区域特征各不相同,但区域气候驱动的环境变化可能会导致区域内湖泊间生物群落变化呈现一致性[20]。青藏高原生态系统长期处于低温环境,生物生长的最适温度低于全球最低值,且当前青藏高原生长季节的温度仍低于其生物生长的最适温度[50]。因此,当前温度上升的幅度和较小的人类活动可能不足以引起硅藻群落的显著变化[24]。

图6硅藻群落PCA分析和多样性指标:所有硅藻物种数据的PCA1、PCA2得分 (两轴解释率分别为6.48%和6.33%)、相对丰度小于5%的硅藻物种的 PCA1(<5%)得分(解释率为5.61%)以及Hill N2和βsor多样性
Fig.6PCA analysis and diversity indicators of diatom communities: PCA1 and PCA2 scores for all diatom species data (6.48% and 6.33% explained by the two axes) , PCA1 (<5%) scores for diatom species with relative abundance <5% (5.61% explained) , and Hill N2 and βsor diversity
虽然硅藻群落整体变化较小,但稀有属种却发生了较为显著的变化。由于优势类群较高的丰度可能会掩盖稀有属种的微妙变化,本研究结果显示必须更加注重对稀有属种变化的监测和分析。理论认为,当生态系统受到外力作用时,稀有属种首先响应,其次是主要类群,最终导致整个生态系统的突变[51]。在过去几十年中出现在该区域LC6湖和尕尔乌克湖中的小型Fragilaria物种被认为是能够适应迅速变化环境的“r-策略者”,这类硅藻属种的出现表明生态系统的高度可变性[24-25,52]。过去几十年来兴伊措硅藻稀有属种相对丰度的下降以及异质性和多样性的上升可能是生态系统对于环境变化响应的早期信号。随着环境压力的进一步增大,该地区湖泊硅藻群落可能会发生显著变化,整个湖泊生态系统可能面临着发生突变的风险[16,53]。
4 结论
过去300年以来,青藏高原东南部的海子山地区受到了气候变化和人类活动的显著影响。本研究发现,1930年之前,湖泊环境和硅藻群落相对稳定,而 1930 年之后,由于气候变化引起的环境变化加剧了兴伊措流域的风化和侵蚀,导致湖泊营养盐增加。人类活动对这一过程可能起到增强作用。自 1930 年以来,湖泊营养盐和pH的变化导致部分硅藻稀有属种的相对丰度出现降低趋势。环境的变化也导致硅藻群落β多样性升高,异质性增强,同时更加多样的生境也使得Hill N2多样性整体呈现上升趋势。本研究强调了在生态系统响应全球变化的研究中关注稀有属种的必要性,因为其变化可能预示着生态系统的突变。